COAGULATION ENHANCEMENT OF AL-GHABAWI LANDFILL LEACHATE USING SEEDS OF MORINGA OLEIFERA
Journal: Water Conservation and Management (WCM)
Husam Al-Hamaiedh, Ahmad Jamrah, A., Shireen Al-Tarawneh, Tharaa M. Al-Zghoul
Print ISSN : 2523-5664
Online ISSN : 2523-5672
This is an open access article distributed under the Creative Commons Attribution License CC BY 4.0, which permits unrestricted use, distribution, and reproduction in any medium, provided the original work is properly cited
Doi: 10.26480/wcm.04.2024.430.443
Abstract
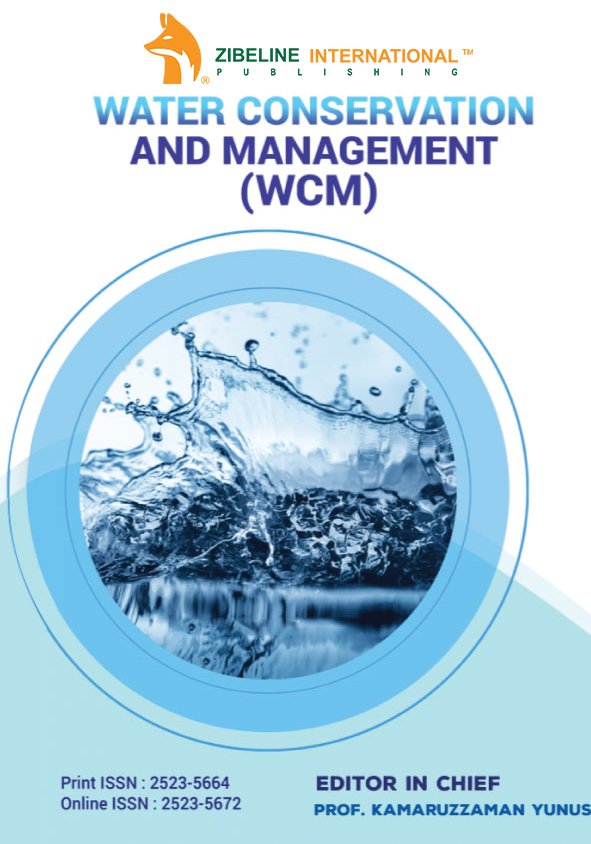
Keywords
Al-Ghabawi landfill, moringa oleifera, alum, landfill leachate.
1. INTRODUCTION
The rapid technological advancement, economic expansion, and urban agglomeration have resulted in a notable increase in the solid waste (SW) generation, which has in turn raised increasing serious concerns for public health and safety across the world (Vergara, and Tchobanoglous, 2012; García-Guaita et al., 2018; Das et al., 2019). According to data from the World Health Organization (WHO), the global urban population was approximately 220 million in 1900, generating less than 300 thousand tons of SW per day (Raghu, and Rodrigues, 2020). By the year 2000, the urban population had risen dramatically to 2.9 billion, leading to the generation of 3 million tons of SW per day. World Bank projections suggest that the annual global generation of SW is expected to increase significantly from 1.3 billion tons to 2.2 billion tons by the year 2025 (Pappu et al., 2007; Elnaklah, and Alotaibi, 2023). All these facts have motivated researchers worldwide to explore effective strategies for solid waste management (SWM) approaches to develop a sustainable environmental system (Raghu, and Rodrigues, 2020; Khan et al., 2024; Alazaiza et al., 2024). The generated SW is divided into agricultural, industrial, domestic waste, and miscellaneous (Abdel-Shafy, and Mansour, 2018). The total volume of municipal solid waste (MSW) generated in the Mediterranean regions, especially in Jordan, has increased from 31.3 million tons in 1980 to 203.6 million tons in 2016, which is equivalent to 2.7 million tons of annual MSW production (Al-Alimi et al., 2022). This makes SWM a global necessity.
The primary operations of SWM include reuse, recycling, composting, and waste prevention, as well as landfilling. Recently many researches have focused on the material recovery of SW as secondary raw materials for industry. A successful reuse of marble and granite sludge as replacements for raw materials in the ceramic industry and as fine aggregate in mortar and concrete was demonstrated by (Al-Hamaiedh, 2010; Al-Hamaiedeh, and Khushefati, 2013; Al-Jrajreh et al., 2023). The study explored the partial replacement of fine aggregate by glass waste (Al-Awabdeh et al., 2022). Additionally, successful incorporation of face masks in concrete mixtures was achieved by Al Swalqah et al, 2023). The organic fraction of municipal SW has been reused for compost production by (Al-Nawaiseh, et al, 2021). Biogas production through the co digestion of sewage sludge and MSW as well as from the organic fraction of MSW has achieved, according to studies by Aljbour et al, 2021; Aljbour, 2021; Al-Hajaya et al., 2021). However, sanitary landfilling is widely recognized as the most extensively utilized in MSW management due to its economic benefits (Babalola and Busu, 2011; Arabeyyat et al., 2024). The waste is usually laid out in thin layers and compacted to minimize its volume as much as possible. It is also regularly covered with suitable material. Throughout this process, numerous biological, physical, and chemical reactions occur, decomposing organic substances. As rainwater percolates through the waste, it combines with these decomposed organic substances, resulting in the creation of a highly polluted liquid referred to as “leachate” (Bilgili et al., 2007; Li et al., 2010; Jamrah et al., 2024). As it drains to bodies of surface water, this leachate may eventually seep into the groundwater and soil (Fan et al., 2006; Wijekoon et al., 2022). High levels of chlorinated inorganic and organic salts, heavy metals (Swar et al., 2023), ammonium nitrogen (Genethliou et al., 2023), and organic matter (OM) (Jotin et al., 2012) can all be found in leachates. Global indices, such as 5-day biochemical oxygen demand (BOD5) (Swar et al., 2023), chemical oxygen demand (COD) (Bouchareb et al., 2022), and total organic carbon (TOC) (Elleuch et al., 2020), are used to define organic pollutants in leachate. The nature of leachate varies significantly among landfills depending on waste composition, climatic conditions (such as rainfall rate), landfill age, and landfilling technology (Abdelaal et al., 2014).
In Jordan, there are 23 landfills; among them, only Al-Ghabawi can be described as a sanitary landfill. As this leachate is not treated, the process is limited to collecting it in open ponds in which it is evaporated, and this method is not environmentally acceptable. However, even in these landfills, the accumulation of untreated leachate is a serious environmental problem (Al-Alimi et al., 2022). Al-Ghabawi landfill is the only sanitary landfill in Jordan and the largest landfill that receives waste from many regions, so it was chosen for this study. The leachate generated from Al-Ghabawi landfill poses a significant threat to the limited water resources in Jordan. This is because the leachate from Al-Ghabawi landfill contains high concentrations of heavy metals (As, Ni, Cd, Mn, and Cr) as well as organic concentrations. The levels of these contaminants exceed water limit standards. On the other hand, the leachate also contains certain heavy metals, which do not have a dangerous effect (Swar et al., 2023; Al, Pb, Zn, and Co). The leachate in Al-Ghabawi landfill is being collected and stored in open lagoons for evaporation (Department of Environmental Studies and Awareness, 2019). Landfill leachate improper disposal poses a serious risk to water and soil ecosystems and is one of the main sources of pollution. It also upsets ecological equilibrium and presents a serious risk to the health of locals (Alazaiza et al., 2024; Maiti et al., 2016; Deng et al., 2020; Keyikoglu et al., 2021). According to reports, there is a chance that very contaminated leachate will seep into the ground and contaminate soils, surface water, and groundwater by (SQ et al., 2015; Bashir et al., 2015). Consequently, before the leachate is released into the environment, it must be properly treated.
Several treatment methods have been employed to treat landfill leachate (LL) with varying degrees of effectiveness (Jamrah et al., 2023). Conventional methods for treating LL have been employed, such as physical, biological, and chemical methods (Mojiri et al., 2021). Chemical treatment processes, including coagulation-flocculation (C/F) and electrochemical treatments such as electro-coagulation (EC), have also been effective (Assou, 2016). Physical treatment processes such as filtration (Da Silva et al., 2014) and adsorption are commonly used to treat LL. Furthermore, a range of biological treatment processes have been effectively studied in both anaerobic and aerobic conditions. Most treatment approaches have limits when it comes to the costly part of funding (Vieira et al., 2010). The chemical treatment process that is commonly used is the coagulation process (Muhammad, M. M., Hamisu, A. J., and Lawan, M. A., 2020). Coagulation, a process for removing suspended and colloidal dissolved particles from water, has proven to be effective and cost-efficient (Muhammad et al., 2020; Chua, 2019). In the coagulation process, chemical and inorganic coagulants are added (Swelam, 2019). These different coagulants can reduce colloidal organic matter, toxic substances, and turbidity (Qureshi, 2016; Rahmadyanti et al., 2021). However, it has a number of drawbacks, including high toxicity, being expensive, producing a lot of sludge, and being environmentally harmful (Qureshi et al., 2016; Al-Qodah et al., 2024). Moreover, sludge produced by chemical coagulants is a source of pollution because it is not biodegradable, which may have a dangerous impact on the environment ( Aal-Hamad, 2023). It has been classified as schedule waste by some countries that require special treatment (Asopa, and Korake, 2019; Hamaideh et al., 2024). Furthermore, synthetic or chemical coagulants are regarded as toxic environmental pollutants as they raise the pH of the water (Rahmadyanti et al., 2021). In addition, Alzheimer’s disease may be brought on by synthetic coagulant residues in water (Nouhi et al., 2019). These reasons have led to an increase in research on the use of natural coagulants, particularly in developing countries.
Several natural materials derived from plants have been studied as potential coagulants for LL treatment. These include Guar gum (Cheng et al., 2020), lateritic soil (Lim, 2012), tannin (Banch, 2019), tamarindus indica seeds (Aziz et al., 2018), and Moringa oleifera (Cao et al., 2021). Moringa oleifera, in particular, has gained global recognition for its medicinal and nutritional value. It belongs to the Moringaceae family, a group of 14 types that comprises shrubs and trees (Banch et al., 2019). The seeds of the Moringa tree have been extensively studied for their coagulation properties (Matouq et al., 2015). The use of Moringa seeds as a coagulant was first documented in 1981, when Sudanese women observed its effectiveness in purifying turbid water from the Nile (Matouq et al., 2015). Previous studies have shown that Moringa oleifera may be used as a coagulant to remove colors, COD, and turbidity (Sivakumar, 2013). Glycerid acid, micro-mineral compounds, polmiric acid, and emulsions found in Moringa oleifera seeds work as chelates to draw metal ions and other particles (Vijayaraghavan et al., 2011). Overall, natural plant-based coagulants offer a promising alternative for LL treatment, and ongoing research aims to explore their effectiveness and potential applications further.
The present paper focuses on conducting a coagulation process to treat leachate production from the Al-Ghabawi landfill by using Moringa oleifera seeds (MOS) as a natural coagulant and alum as a commercial coagulant. These experiments were conducted in several stages. The first stage involved adding the same amount of alum to find the optimum pH that achieved the best removal of turbidity. The next stage was adding different doses of alum at the optimum pH, then the best dose of alum was determined. After that, the coagulation process was performed by adding different doses of Moringa seeds with the optimum dose of alum at the optimum pH, and then the best dose of Moringa seeds was determined. Finally, the experiment was carried out using Moringa seeds alone as a natural coagulant at the optimum pH and determining the best doses of Moringa seeds. The study aims to explore the optimum parameters of the coagulation process of leachate treatment by using the seeds of Moringa oleifera as a natural coagulant and alum as a commercial coagulant, assess the performance of the coagulation process in the removal of the main pollutants from the leachate, and evaluate the quality of the treated leachate and the potential of its reuse.
2. DESCRIPTION OF THE STUDY AREA
The Al-Ghabawi landfill is situated in the eastern desert at Uhud, 40 kilometers from Amman. Situated at (31° 55’44.0″ N and 36° 10’56.0 E), the property covers an approximate area of 2 km2, including semi-flat terrain with an average gradient of 1.4% from southeast to northwest. The closest residential area is located almost 7 km to the southwest and west of the landfill; the surrounding area is characterized as a semi-arid desert with poor soil quality and low organic content, particularly in the eastern and southern regions. The Amman Municipality is not the only location served by the landfill; in addition to the capital, Amman, other regions served by the landfill include the municipalities of the army, Rusaifa, Zarqa, Na’ur, Sahab, Muwaqqar, the private sector, and others (Yamin, 2017).
The Al-Ghabawi landfill is Jordan’s first of its sort. Gas collection systems were included in the landfill’s construction and design. Since 2003, waste has been disposed of in cells that make up the landfill (Yamin, 2017). Four years after the landfill’s establishment, in 2007, a system for gathering leachate was installed inside the landfill . Each landfill cell has a submersible pump inside it that pumps the leachate toward the succulent basins as part of the leachate system. To protect the surrounding ecosystem, these basins have been designed and coated with insulating materials to stop leachate from leaking into the soil and groundwater .
As seen in Figure 1, the leachate is collected in ponds and allowed to evaporate naturally. It was intended to treat leachate through final infiltration, sedimentation, and an aeration system (surface aerators). But as of right now, the treatment system is not operative. With a maximum evaporation surface of 19,000 m2, the current maximum capacity for leachate storage is about 44,000 m3. Leachate generation peaks at around 445,606 m3/y, or about 5090 m3/h on a daily average.
3. MATERIALS AND METHODS
3.1 Materials
3.1.1 Preparation of A Leachate Sample
Leachate samples were collected from the Al-Ghabawi landfill located in the eastern desert near Uhud from March 2019 to October 2020. Raw samples were collected from the outflow box in Cell #5, as shown in Figures 2a and b. Samples of leachate were taken from the midpoint of the leachate depth. Throughout the sample process, leachate was completely filled into each sampling container without any air bubbles. A 10-liter sample was collected, and it was stored in a polyethylene container before being transported to the laboratory and kept at 4 ˚C as described to be used for two consecutive days as suggested by the Standard Methods of Chemical Analysis and the standard conservation methods for the examination of water and wastewater (APHA Awwa, 2005; Kulikowska and Klimiuk 2008; Tatsi et al., 2003). This is usually carried out to reduce the possibility of biodegradation or volatilization. Because the leachate is a concentrated liquid—which has a dark black color—it was diluted with distilled water to be used in jar tests. The leachate samples were diluted by adding 800 ml of distilled water to every 200 ml of leachate and stirring for about 60 seconds.
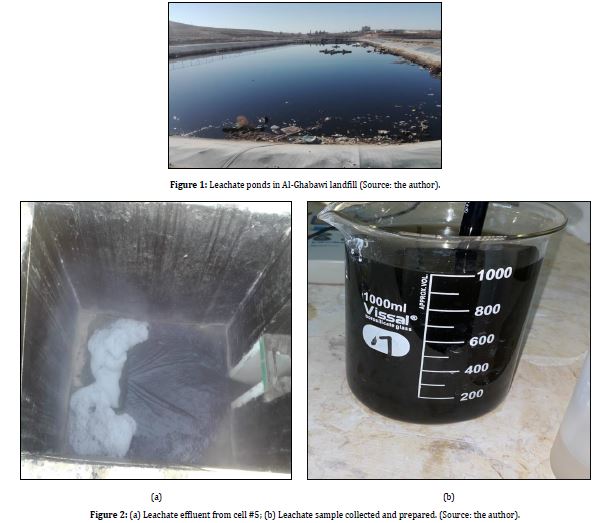
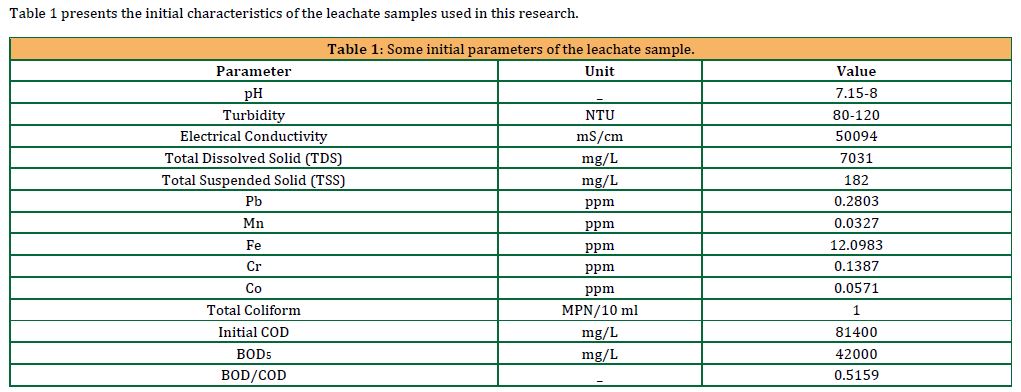
3.1.2 Preparation of Moringa Oleifera Seeds
The Moringa oleifera used in this study was obtained from the governorate of Irbid, and it is of Sudanese origin. It was formed as a plant from which one kilogram of seeds was extracted, as shown in Figures 3a and b. According to seeds were then dried in an oven (Memmert, Gemini Sustainable Lab Equipment, The Netherlands) at 50 ˚C for a whole day in order to produce extract for use in treatability studies. After that, the wings of the seeds were manually removed using a knife since, according to unshelled Moringa seeds produce the greatest outcomes when compared to shelled seeds. After that, as suggested the seeds were placed in a food blender to obtain powdered moringa seeds, as shown in Figure 3c by Katayon et al.2006). The powder was then sieved using a 0.75-mm sieve, as recommended by (Sivakumar, 2013). Then, in a beaker, 10 g of powdered seeds were dissolved in 1000 ml of distilled water. The combination was homogeneously mixed for two minutes at high speed using a food blender. After that, the combination was kept at 5 ˚C for a whole day. The solution was then used for the coagulation process.
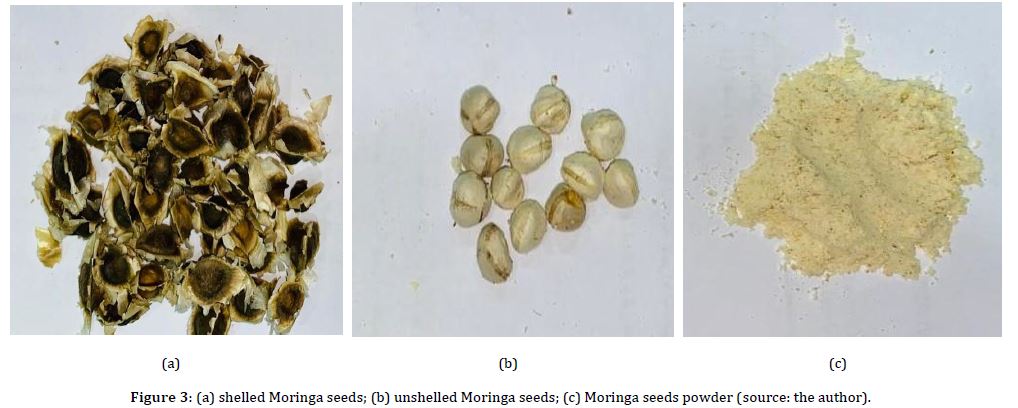
3.1.3 Chemicals
All chemicals used in this study were of analytical grade as recommended by the AEEP Environmental Engineering Unit operation and unit process laboratory manual. The chemicals, including sodium hydroxide NaOH (0.1 M), sulfuric acid H2SO4 (0.05 M and 0.02 M), and aluminum sulfate Al2(SO4)3.18H2O (10,000 mg/L), were bought from Alnoorien Company.
3.2 Methods
3.2.1 Jar Test
In order to conduct this test, a number of water samples must be prepared and put in a multi-stirring device (Stuart Scientific, UK). The samples are then calibrated with a variable range of coagulant salts: 10, 15, 20, 25, 30, 35, 40, 45, 50, 55, 60, and 65 mg/L. The samples are then vigorously stirred with a glass rod and allowed to settle for 30 minutes. After that, the water is allowed to calm down and settle for an entire hour. The water, which is described as ultra-floaty, is then examined to determine its color and degree of turbidity. In this case, the lowest amount of coagulated salts can be estimated and evaluated to provide adequate removal for the intended purpose.
The second set of samples is prepared with a pH degree above the usual range of 4, 4.5, 5, 5.5, 6, 6.5, 7, 7.5, 8, 8.5, and 9. The amount of previously coagulated salt is determined and added to each beaker, where it is stirred, aggregation, and merging. Sedimentation and fine particles. It is feasible to verify the proper pH and examine the floating materials. If required, the amount of coagulated salt can be adjusted.
3.3 Pre-Treatment
3.3.1 Determination of Optimum pH
Six beakers were filled with one liter of leachate prepared at varied pH values of 4, 4.5, 5, 5.5, 6, 6.5, 7, 7.5, 8, 8.5, and 9 in order to examine the optimum leachate pH for the coagulation process. Through the addition of H2SO4 and NaOH solutions, the samples’ pH was adjusted. The samples were measured using a pH meter (Senso Direct 150) to get exact pH readings. The samples were placed at the intensity of rapid mixing at 100 rpm for two minutes, and aluminum sulfate was added in two ranges: 20 mg/l and 200 mg/l, to each beaker. After two minutes of rapid mixing, followed by 15 minutes of slow mixing at 30 rpm. The beakers are then gently taken out, and their stability and sedimentation are examined. After, samples were taken at the 30 minute, 60 minute, and 90 minute sedimentation times. 10 ml were then taken in the middle of each beaker by pipettes, the turbidity was measured by a (portable turbidity meter 430-260) and the optimal pH was determined.
3.3.2 Determination of The Optimum Dosage Of Alum
In this experiment, 1000 ml of leachate was used to determine the optimum dosage of alum. Optimum pH was sustained throughout the experiment with the addition of either 0.1 M NaOH or (0.05 M and 0.02 M) H2SO4 solution. Then, adding different amounts of alum solution in two ranges (10, 20, 30, 35, 40, 45, 50, 55, 60, 70, 80, 90 mg/l) and (100, 200, 300, 400, 500, 600, 700, and 800 mg/l) to the beakers coincided with the operation of the device. With a rapid mix velocity of 100 rpm for two minutes and then slow mixing at 30 rpm for 15 minutes, the sample was left for stability at sedimentation times of 30 min, 60 min, and 90 min. Then taking 10 ml of the leachate sample at the intermediate depth of the beaker by pipettes. Hence, the turbidity was measured for each sample, and the optimal dose of alum was determined from two ranges. After that, the optimal sample was taken, and several parameters were analyzed; total suspended solid (TSS), total dissolved solid (TDS), electrical conductivity (EC), COD, BOD, and atomics (Cr, Fe, Mn, Pb, and Co).
3.3.3 Determination of the Optimum dosage of Moringa Oleifera
After finding the optimum pH and the optimum dosage of alum, we used them for this experiment to find the optimum dosage of Moringa oleifera. The beakers were filled with leachate, the optimum pH was determined, and the optimum dose of coagulant was added to all samples. After that, various amounts of Moringa oleifera were added that were previously prepared by two ranges (10, 20, 30, 35, 40, 45, 50, 60, and 70 mg/l) and (100, 150, 200, 250, 300, 400, 500, 600, and 700 mg/l), with a rapid mix velocity of 100 rpm for two minutes, then slow mixing at 30 rpm for 15 minutes. The sample was left for stability at sedimentation times of 30 min, 60 min, and then taking 10 ml of the sample from the middle of each beaker. The turbidity was measured for each sample, and the optimal dosage of Moringa oleifera was determined for two ranges. After that, the optimal sample was taken, and several analyses were made: TSS, TDS, EC, COD, BOD, and atomics (Cr, Fe, Mn, Pb, and Co).
3.3.4 Determination of the Optimum Dosage of Moringa Oleifera Alone as a Coagulant
After determining the optimal pH and the optimum dose of Moringa oleifera seeds, Moringa oleifera seeds were used alone as a coagulant. beakers were prepared and filled with the pre-prepared leachate, and the optimum pH was adjusted for all of them. The jar test device was turned on, and the pre-prepared Moringa solution was added with doses of (10, 20, 30, 35, 40, 45, 50, 60, 70, and 80 mg/l) and (100, 200, 300, 400, 500, 600, 700, and 800 mg/l), with a rapid mix velocity of 100 rpm for two minutes, then slow mixing at 30 rpm for 15 minutes. The sample was left for stability at sedimentation times of 30 min, 60 min, then taking 10 ml of the sample from the middle of each beaker. The turbidity was measured for each sample, and the optimal dosage of Moringa oleifera alone was determined for two ranges. After that, the optimal sample was taken, and several parameters were analyzed: TSS, TDS, EC, COD, BOD, and atomics (Cr, Fe, Mn, Pb, and C).
3.4 Analytical
Samples were analyzed before and after each treatment stage, where the samples to be measured for heavy metals, including Pb, Mn, Co, Fe, and Cr, were filtered using a 0.45μm syringe filter (Standard Method 3111 B). EC was measured using the CON 6-LaMotte instrument (LaMotte Co., Washington, USA), with the values reported in mS/cm. Several physico-chemical parameters, including turbidity used (Standard Method 2130A) by VEP SCIENTIFICA TB1, Italy, pH used (Standard Method 4500-H+B), TSS used (Standard Method 2540 D), TDS used (Standard Method 2540 C), total coliform used (Standard Method 9223 B), E. coli used (Standard Method 9223 B), COD used (Standard Method 5210 D), and BOD used (Standard Method 5220 D) for leachate were carried out at Prince Faisal Center for Research on the Dead Sea, Environment, and Energy (APHA Awwa, 2005).
The percentage removal efficiency (R%) was calculated according to the following equation (Castañeda-Díaz et al., 2017): (10)
Where C0 and Ce are the initial and final COD concentrations (mg/L) of each of the parameters (turbidity, COD, and BOD).
4. RESULTS AND DISCUSSIONS
4.1 The Effect of Turbidity
The leachate was coagulated using alum and Moringa oleifera seeds as coagulants. The turbidity changed as a function of time, and the experiment’s findings were used to calculate the optimum pH, alum dosage, and Moringa oleifera seed dosage.
4.1.1 Effects of pH on Turbidity and Determining the Optimum pH
The coagulation process is highly dependent on pH, whereas the water’s pH effects the coagulants’ surface charge, which in turn effects the suspension’s level of stability (Oladoja, 2015). Moreover, it effects the effectiveness of the coagulation process ( Lester-Card et al., 2023). In this study, the impact of the leachate’s initial pH (which ranged from 7.15 to 8) was examined to determine the optimum pH for maximum efficiency. Figure 4 shows the results of the removal efficiency of turbidity due to the coagulation process with 20 mg/L of alum, as affected by the pH values ranging from 4 to 9. Additionally, the removal efficiencies of turbidity shown are for sedimentation times of 30 min, 60 min, and 90 min.
Based on the results illustrated in Figure 4, the turbidity removal efficiency increases with increasing pH values and decreases with increasing sedimentation times. At a pH range of 6 to 9, the removal percentages of turbidity decreased, ranging from 10% to 7.5%, 7.5%, and 6.67% for 30, 60, and 90 minutes, respectively. In contrast, from pH 4 to 5, the removal percentages of turbidity were negative, which indicates that the alum is usually in a dissolved state at these pH levels. Hence, not only coagulation does not take place, but also dissolved alum contributes to the turbidity. Furthermore, the percentage removal of turbidity at pH 5.5 is the highest across the pH range, with reductions of 15.833%, 15%, and 15% after 30, 60, and 90 minutes, respectively (Lester-Card et al.,2023). Found that the alum coagulant operated best at pH values between 5 and 9. The optimum pH of 5.5 was considered in the consecutive determination of the optimum coagulant dosage for turbidity removal in leachate.
After that, we increased the dose of alum from 20 mg/L to 200 mg/L. Figure 5 shows the results of the removal efficiency of turbidity due to coagulation with 200 mg/L of alum, as affected by the pH values ranging from 4 to 9. The turbidity values shown are for sedimentation periods of 30, 60, and 90 minutes.
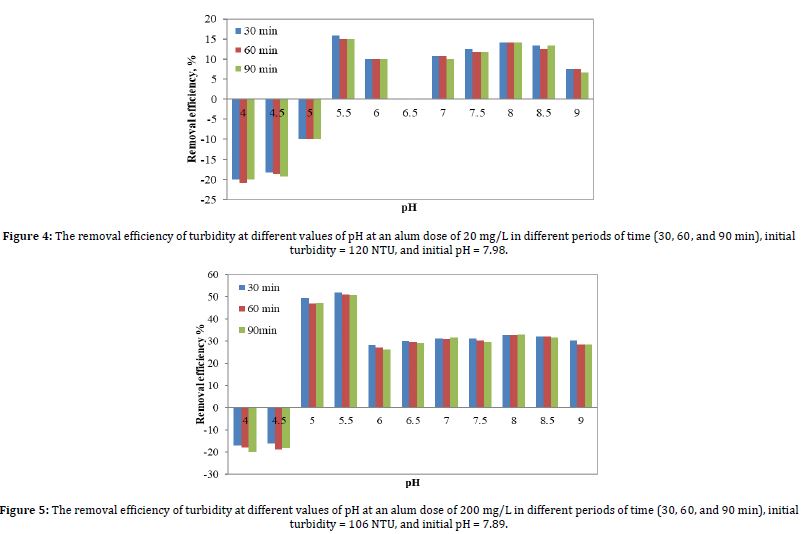
As shown in Figures 4 and 5, the percentages of removing turbidity from leachate differed significantly when the alum dose was increased from 20 mg/L to 200 mg/L, as the removal efficiency increased from 15.83% to 51.88% for the alum dose of 20 mg/L and 200 mg/L, respectively, at pH 5.5 and sedimentation time of 30 minutes. In addition to the differences, the pH ranges differed at the results of the negative removal efficiency of turbidity, as the values of the negative removal percentages ranged at the pH from 4 to 5 and 4 to 4.5, at the alum dose of 20 mg/L, and 200 mg/L, respectively.
Based on the results illustrated in Figure 5, the percentage of turbidity removal decreases with increasing pH values. At a pH range of 5.5 to 9, the removal percentages of turbidity decreased, ranging from 51.88%, 50.94%, and 50.94% to 30.19%, 28.3%, and 28.3% for 30, 60, and 90 minutes, respectively. This is explained by the sluggish, extremely weak reactions that happen at high pH levels. In contrast, from pH 5 to 5.5, the turbidity removal efficiencies were very high, within the range of 49.33% to 51.88%. Within the pH range of 4 to 5.5, there was a significant improvement in the effectiveness of removing turbidity. With a 51.88% removal rate at 30 minutes, pH 5.5 has the best turbidity removal effectiveness across the pH range. This is far more than the mere -17% elimination at pH 4.
4.1.2 Effect of Alum Doses on Turbidity at Optimum Ph 5.5 And Determined the Optimum Alum Dose
The effect of alum dosage on the turbidity removal in leachate was examined by varying the alum dosages between 10 and 80 mg/L. In each of the jar test experiments, the pH of the leachate samples was set at an optimum 5.5. Following the test, the samples were allowed to settle for 30, 60, and 90 minutes before the turbidity measurement was conducted. Figure 6 shows the results of the removal efficiency of turbidity due to coagulation when the optimum pH value was 5.5 as effected by alum doses.
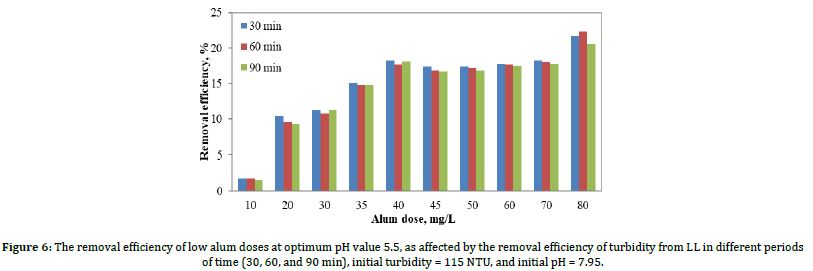
As shown in Figure 6, the best removal efficiency was achieved when the alum dose was 40 mg/L. Where the removal when alum doses was 45, 50, 60, 70, and 80 mg/L was very close to 40 mg/L, but the 40 mg/L were chosen in terms of cost and quantity. Beyond this dose, no additional alum dosage has made a discernible difference in the removal efficiency. The plotted graph in Figure 6 shows that the best percentage of turbidity removal (18.26%) was obtained at 40 mg/L of alum dosage, which is more than the lowest dosage (10 mg/L) with only 1.74% of turbidity removal.
After that, we increased the dose of alum from 80 mg/L to 800 mg/L. Figure 7 shows the results of the removal efficiency of turbidity due to coagulation when the optimum pH value was 5.5 as effected by alum doses. Alum doses ranged from 100 to 800 mg/L; the turbidity values shown are for sedimentation periods of 30, 60, and 90 minutes.
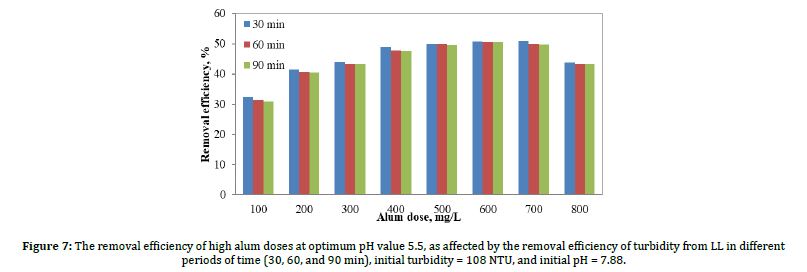
As shown in Figure 7, the best removal efficiency was achieved when the alum dose was 400 mg/L. Where the removal when alum doses was 500, 600, and 700 mg/L, very close to 400 mg/l, but the 400 mg/L were chosen in terms of cost and quantity. Additionally, the figure indicates a decrease turbidity removal efficiency at 800 mg/L of alum; this can be taken as evidence that the predominant coagulation mechanism is charge neutralization. Furthermore, the best percentage removal of turbidity occurred at 400 mg/L of alum dosage, with turbidity removal efficiencies of 48.9%, 47.9%, and 47.7% after 30, 60, and 90 minutes, respectively.
4.1.3 Effects of Optimum Alum Dose and Moringa Oleifera Seed Doses on Turbidity at Optimum pH 5.5 and Determined the Optimum Moringa Dose
After determining the optimum pH value was 5.5 and also the optimum removal of turbidity at 40 and 400 mg/l of alum, with a removal efficiency of 18% and 48%, respectively, Figure 8 shows the results of the removal efficiency of turbidity due to coagulation when the optimum pH value was 5.5 and the optimum alum dose was 40 mg/L, as affected by Moringa seed doses. Moringa seeds ranged from 10 to 70 mg/L, the turbidity values shown are for sedimentation times of 30 min, 60 min, and 90 min.
According to Figure 8, the best removal efficiency was achieved when the alum dose was 40 mg/l. The removal of Moringa seed doses was 45, 50, 60, and 70 mg/l, very close to 40 mg/l, but the 40 mg/l were chosen in terms of cost and quantity.
Figure 9 shows the results of the removal efficiency of turbidity due to coagulation when the optimum pH value was 5.5 and the optimum alum dose was 400 mg/l, as affected by Moringa seed doses. Moringa seeds ranged from 100 to 700 mg/l, the turbidity values shown are for sedimentation times of 30 min, 60 min, and 90 min.
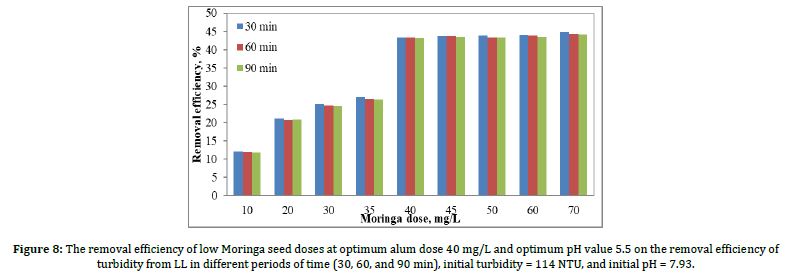
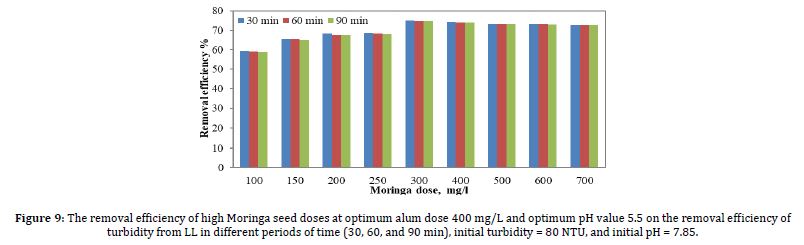
According to Figure 9, the best removal efficiency was achieved when the alum dose was 300 mg/l. The removal of Moringa seed doses was 400, 500, 600, and 700 mg/l, very close to 300 mg/l, but the 300 mg/l were chosen in terms of cost and quantity
4.1.4 Effect of Moringa Seeds Alone as A Coagulant on Turbidity and Determined The Optimum Dose Of Moringa Seeds
The coagulation experiment was carried out using Moringa seeds alone as a natural coagulant. Figure 10 shows the results of the removal efficiency of turbidity due to coagulation when the optimum pH value was 5.5 as affected by Moringa seeds. Moringa seed doses ranged from 10 to 80 mg/l; the turbidity values shown are for sedimentation times of 30 min, 60 min, and 90 min.
According to Figure 10, The best removal efficiency was achieved when the alum dose was 45 mg/l. Where the removal when alum doses was 50, 60, 70, and 80 mg/l was very close to 45 mg/l, but the 45 mg/l were chosen in terms of cost and quantity.
Figure 11 shows the results of the removal efficiency of turbidity due to coagulation when the optimum pH value was 5.5 as affected by Moringa seeds. Moringa seed doses ranged from 100 to 800 mg/l; the turbidity values shown are for sedimentation times of 30 min, 60 min, and 90 min.
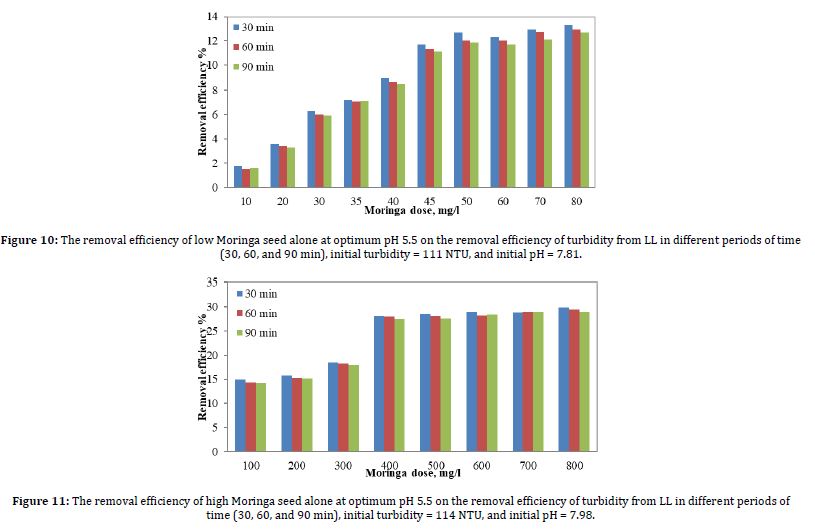
According to Figure 11, the best removal efficiency was achieved when the alum dose was 400 mg/l. Where the removal when alum doses were 500, 600, 700, and 800 mg/l was very close to 400 mg/l, but the 400 mg/l were chosen in terms of cost and quantity.
Whereas, there was no significant effect on the rate of turbidity removal when using Moringa seeds alone as a coagulant compared to when using Moringa dose and alum dose together as coagulants, as their results were better.
4.2 Effect On TSS Removal
Suspended solid particles in water and wastewater cause scattering of light, leading to turbidity in the water (Rügner et al., 2013). Solid particles that are soluble in water, both organic and inorganic, make up TSS. Excessive levels of TSS in water bodies can disturb aquatic life and cause sedimentation (Azis et al., 2015). Therefore, it is expected that the behavior of suspended solid particles will be similar to that of turbidity in the landfill leachate. Figure 12 shows the results of the removal efficiency of TSS due to coagulation when the optimum pH value was 5.5, the optimum alum dose, and the optimum Moringa seed dose.
The results of this study, as shown in Figure 12, show that the addition of alum alone led to the lowest removal efficiency of TSS, amounting to 9.89% and 16.48% for low and high doses, respectively. On the other hand, the addition of Moringa oleifera alone slightly improved the removal efficiency of alum alone, reaching 16.48% and 17.03% for low and high doses, respectively. Figure 4 shows that the TSS removal efficiency is improved when optimum Moringa dose is added, better than when the alum dose is added. The instability of negatively charged colloids in cationic polyelectrolytes was the reason for Moringa oleifera removal of TSS (Chaouki et al., 2017) .
In contrast, the maximum TSS removal efficiency was achieved by adding the optimum amount of alum and Moringa oleifera to the coagulation process, at 41.21% and 33.52% for high and low dosages, respectively. This result is due to the addition of alum and Moringa oleifera, which effect hydrolysis products, and cations interact with negatively charged colloids to cause charge neutralization. There is a decrease in suspended particles as neutral solids sediment to the bottom due to gravity (Ramprasad et al., 2019; Gautam and Saini, 2020).
4.3 Effect on TDS Removal
Figure 13 shows the results of the removal efficiency of TDS due to coagulation when the optimum pH value was 5.5, the optimum alum dose, and the optimum Moringa seed dose. To see which of these stages yields the best removal, the alum dose and Moringa seed dose were added together as coagulants for each dose.
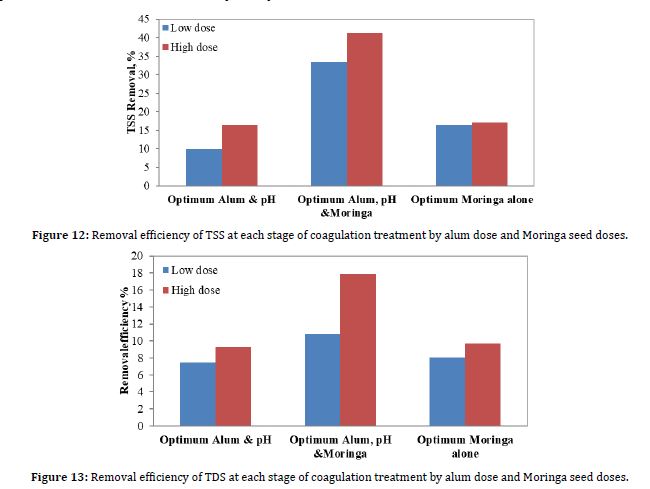
According to Figure 13, the best removal efficiency was achieved when the alum dose and Moringa dose were added together, reaching 17.9% and 10.81% for high and low doses, respectively. The result shows that the TDS removal efficiency is improved when the optimum Moringa dose is added, better than when the alum dose is added. There was no significant effect on TDS, as elimination rates were very low for all stages.
4.4 Effect on EC Removal
Table 2 shows the EC value at each stage of the coagulation treatment process by alum and Moringa oleifera seeds at optimum doses.
Table 2 illustrates the effect of coagulant type on leachate conductivity. The effluent’s conductivity was 50094 mS/cm prior to treatment. Following the treatment procedure, it was discovered that the conductivity values for employing alum alone, alum and Moringa oleifera combined, and Moringa oleifera alone were decreased to 10695, 10352, and 10674 mS/cm, respectively. This may be the result of the coagulants ability to conduct electricity due to a decrease in dissolved ions.
Figure 14 shows the results of the removal efficiency of EC due to coagulation when the optimum pH value was 5.5, the optimum alum dose, and the optimum Moringa seed dose.
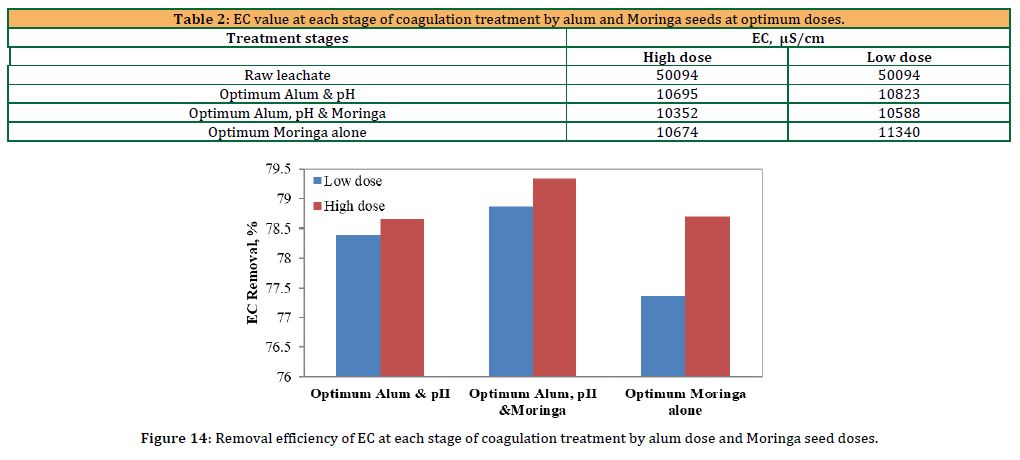
According to Figure 14, the best removal efficiency was achieved when the alum dose and the Moringa dose were added together. The figure shows that the removal efficiency was large and close for all stages.
4.5 Effect on Heavy Metals Removal
Generally, low concentrations of heavy metals are observed. Human health may be impacted by high concentrations of heavy metals (Shan et al., 2017). Thus, before being released into the environment, heavy metals need to be treated. The concentrations of heavy metals in the different treatment steps are presented below.
4.5.1 Effect on Iron (Fe) Removal
Figure 15 shows the results of the removal efficiency of Fe due to coagulation when the optimum pH value was 5.5, the optimum alum dose, and the optimum Moringa seed dose. To see which of these stages yields the best removal, the alum and Moringa seed doses were added together as coagulants for each dose.
According to Figure 15, the best removal efficiency was achieved when the alum dose and the Moringa dose were added together. In Figure 15, the coagulation process with Moringa oleifera is able to remove Fe up to 87.9% and 74.52% for high and low doses, respectively, while the addition of alum slightly improved the removal of Fe, reaching 92.21% and 83.3% for high and low doses, respectively. This result shows that the Fe removal efficiency is improved when an optimum alum dose is added, rather than when optimum Moringa seeds are added alone. These results indicate the effectiveness of the coagulation process when using alum and Moringa oleifera together as coagulants.
4.5.2 Effect on Lead (Pb) Removal
Figure 16 shows the results of the removal efficiency of Pb due to coagulation when the optimum pH value was 5.5, the optimum alum dose, and the optimum Moringa seed dose. To see which of these stages yields the best removal, the alum and Moringa seed doses were added together as coagulants for each dose.
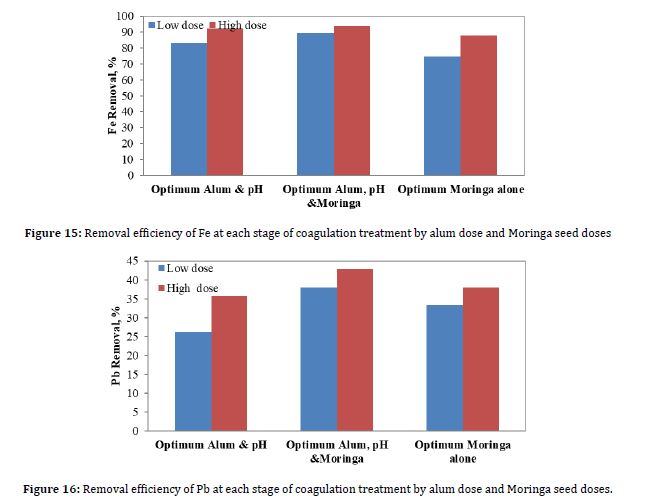
According to Figure 16, the best removal efficiency was achieved when the alum dose and the Moringa dose were added together, reaching 38% and 42.8% for the low and high doses, respectively. The figure shows that the Pb removal efficiency is improved when the optimum Moringa dose is added, rather than when the optimum alum is added. In contrast to the Fe removal results, only the coagulation process achieved a lower removal efficiency for pb compared to Fe. In addition, and in contrast to the results for Fe, using Moringa oleifera as a coagulant achieved better lead removal efficiency than using alum as a coagulant.
4.5.3 Effect on Manganese (Mn) Removal
Figure 17 shows the results of the removal efficiency of Mn due to coagulation when the optimum pH value was 5.5, the optimum alum dose, and the optimum Moringa seed dose. To see which of these stages yields the best removal, the alum and Moringa seed doses were added together as coagulants for each dose.
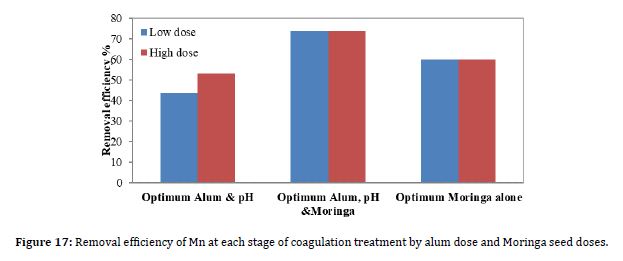
According to Figure 17, the best removal efficiency was achieved when the alum dose and the Moringa dose were added together. The figure shows that the Mn removal efficiency is improved when the optimum Moringa dose is added, rather than when the optimum alum is added.
4.5.4 Effect on Chromium (Cr) Removal
The Cr causes various threats to humans and animals, such as cancer and mutations. It is also easily transported into the environment due to its ability to react and dissolve in water (Öman et al., 2000). Figure 18 shows the results of the removal efficiency of Cr due to coagulation when the optimum pH value was 5.5, the optimum alum dose, and the optimum Moringa seed dose. To see which of these stages yields the best removal, the alum and Moringa seed doses were added together as coagulants for each dose.
According to Figure 18, the best removal efficiency was achieved when the alum dose and the Moringa dose were added together.
4.5.5 Effect on Cobalt (Co) Removal
Figure 19 shows the results of the removal efficiency of Co due to coagulation when the optimum pH value was 5.5, the optimum alum dose, and the optimum Moringa seed dose. To see which of these stages yields the best removal, the alum and Moringa seed doses were added together as coagulants for each dose.
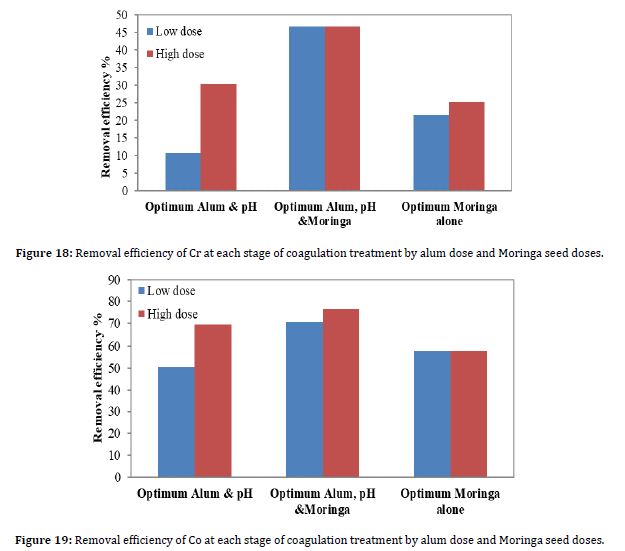
According to Figure 19, the best removal efficiency was achieved when the alum dose and the Moringa dose were added together. There was no significant difference between the coagulation stages, as the results were somewhat similar.
4.6 Effect on COD Removal
The COD represents the amount of oxygen required to completely oxidize the organic waste constituents chemically to inorganic end products (Akcin et al., 2005). The COD value for the leachate sample from the landfill site was 81400 mg/L. Figure 20 shows the results of COD removal efficiency due to coagulation when the optimum pH value was 5.5, the optimum alum dose, and the optimum Moringa seed dose. To see which of these stages yields the best removal, the Moringa seed dose was added as a coagulant for each dose.
The results showed that the use of Moringa oleifera and alum together as coagulants was able to produce COD removal efficiency of 88% and 87.92% for high and low doses, respectively, while alum reached an efficiency of 80.2% and 76.17% for high and low doses, respectively, as shown in Figure 20. According to Figure 20, the best removal efficiency was achieved when Moringa seed alone was added. The figure shows that the COD removal efficiency is improved when the optimum Moringa dose and the optimum alum dose are added, rather than when the optimum alum dose is added.
After the addition of Moringa oleifera seeds to the prepared leachate, the initial COD decreased. The COD was reduced by 91.57% and 88.14% for high and low doses, respectively. The reduction was wide-ranging, from 81400 mg/L before treatment to 6860 and 9650 mg/L after treatment with high and low Moringa oleifera doses, respectively. This suggests that Moringa oleifera, because it decreases solids, nutrients, and organics, is a suitable coagulant for COD reduction. (Patel and Vashi, 2013; Suhartini et al., 2013) reported that Moringa oleifera reduced wastewater COD.
4.7 Effect on BOD Removal
The BOD is the measure of the biodegradable organic mass of leachate, and that indicates the maturity of the landfill, which typically decreases with time (Bhalla et al., 2013; Al-Zghoul et al., 2023). In this study, the BOD value for the raw leachate was 42000 mg/L. Figure 21 shows the results of BOD removal efficiency due to coagulation when the optimum pH value was 5.5, the optimum alum dose, and the optimum Moringa seed dose. To see which of these stages is the best removal, the best result was achieved when Moringa seed was added as a coagulant for each dose.
The results showed that the use of Moringa oleifera and alum together as coagulants was able to improve BOD removal efficiency by 80.95% and 71.43% for high and low doses, respectively, while alum reached an efficiency of 61.9% and 57.14% for high and low doses, respectively, as shown in Figure 21. According to Figure 21, the best removal efficiency was achieved when Moringa seeds alone were added. The figure shows that the BOD removal efficiency is improved when the optimum Moringa dose and the optimum alum dose are added, rather than when the optimum alum dose is added.
After the addition of Moringa oleifera seeds to the prepared leachate, the initial BOD decreased. The BOD was reduced by 85.71% and 80.95% for high and low doses, respectively. The reduction was wide-ranging, from 42000 mg/L before treatment to 6000 and 8000 mg/L after treatment with high and low Moringa oleifera doses, respectively.
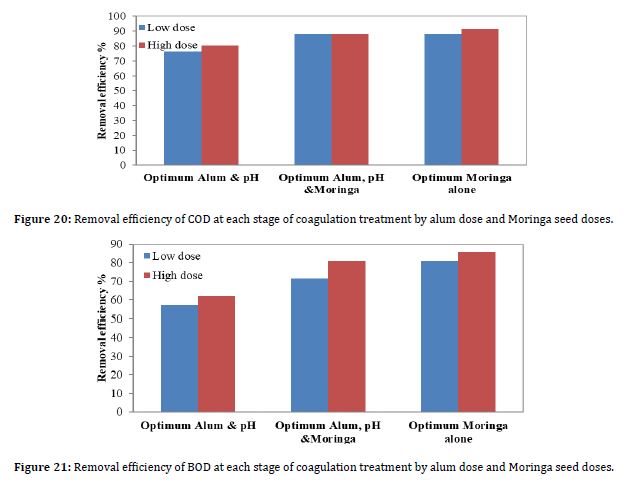
5. CONCLUSIONSBased on the findings of this study, the following conclusions can be drawn:
• The best removal of turbidity was achieved at an optimum pH of 5.5.
• It was found that the use of Moringa seeds with alum doses together as coagulants gave the best removal of turbidity, followed by the use of alum alone as a coagulant, which gave better removal of turbidity than the seeds of Moringa alone.
• The TSS was removed at a moderate rate; the best removal of TSS was when Moringa seeds and alum doses were used together as coagulants, followed by Moringa seeds alone, then alum alone.
• The TDS was removed at a low rate; the best removal of TDS was when Moringa seed dose and alum dose were used together as coagulants.
• The results showed that the heavy metals (Fe, Pb, Mn, Cr, and Co) that were analyzed achieved the best removal when using Moringa seed dose and alum dose together as coagulants. The best removal was by a high percentage of iron, followed by cobalt, manganese, chromium, and lead, respectively.
• It achieved the best removal efficiency of COD and BOD by using Moringa seeds alone as a coagulant with high percentages, followed by Moringa seeds and alum doses together as coagulants, and then alum alone.
• Moringa oleifera was able to enhance the coagulation process with alum. This was evidenced by a significant decrease in the parameters.
• Moringa oleifera seeds can be used as a natural coagulant to reduce the COD and BOD of LL.
• The results indicated that the use of Moringa oleifera as a coagulant for removing BOD in a MSW leachate seems to be an economical and worthwhile alternative over conventional methods.
RECOMMENDATIONS
Future researchers are recommended to consider the following recommendations based on the findings of this study:
• Developing the leachate treatment plant in Al-Ghabawi landfill to make it feasible and operating it in the landfill, as the process in the landfill is limited to collecting it in ponds and evaporating it.
• Starting to improve the treated LL to be used for irrigation purposes and to make use of it in one way or another.
• Conducting more research related to the use of Moringa oleifera on other types of leachate with different concentrations and properties.
• Doing experiments on LL using other natural materials.
• Carrying out more research and studies related to the effectiveness of treatment with Moringa and other coagulants and in the presence of other biological, physical, or chemical treatment techniques.
• Doing a biological treatment of the leachate to increase the efficiency of organic matter, ammonia, and other materials.
REFERENCES
Aal-Hamad, K. K., 2023. Optimization Of The Performance Of Date Seed As a Natural Coagulant For Industrial Wastewater Treatment (Doctoral dissertation).
Abdelaal, F.B., Rowe, R.K., Islam, M.Z., 2014. Effect of leachate composition on the long-term performance of a HDPE geomembrane. Geotext. Geomembr. 42, Pp. 348–362.
Abdel-Shafy, H. I., and Mansour, M. S., 2018. Solid waste issue: Sources, composition, disposal, recycling, and valorization. Egyptian journal of petroleum, 27(4), Pp. 1275-1290.
Akcin, G., Alp, Ö., Gulyas, H. and Büst, B., 2005. Characteristic, analytic and sampling of wastewater. EMWater E-Learning Course, Lesson A, 1, Pp.1-49.
Al Swalqah, R. A. A., Al-Kheetan, M. J., Jweihan, Y. S., and Al-Hamaiedeh, H., 2023. Synergistic Effect of Treated Polypropylene-Based Disposable Face Masks on Durability and Mechanical Properties of Concrete. Arabian Journal for Science and Engineering, Pp. 1-9.
Al-Alimi, A.K.A., Al-Sarheed, H.T., Elarabiyat, O., and Rimawi, O.A., 2022. Heavy Metals and PAHs in the Municipal Solid Waste in Ghabawi Landfill/Jordan.
Al-Awabdeh, F. W., Al-Kheetan, M. J., Jweihan, Y. S., Al-Hamaiedeh, H., and Ghaffar, S. H., 2022. Comprehensive investigation of recycled waste glass in concrete using silane treatment for performance improvement. Results in Engineering, 16, 100790.
Alazaiza, M. Y., Alzghoul, T. M., Al Maskari, T., Amr, S. A., and Nassani, D. E., 2024. Analyzing the Evolution of Research on Student Awareness of Solid Waste Management in Higher Education Institutions: A Bibliometric Perspective. Sustainability, 16(13), 5422.
Al-Hajaya, M., Aljbour, S. H., Husam, A. H., Abuzaid, M., Tayel, E. H., Hemidat, S., and Nassour, A., 2021. Investigation of energy recovery from municipal solid waste: a case study of Al-karak city/Jordan. Civil and Environmental Engineering, 17(2), Pp. 610-620.
Al-Hamaiedeh, H. D., and Khushefati, W., 2013. Granite sludge reuse in mortar and concrete. Journal of Applied Sciences, 13(3), Pp. 444-450.
Al-Hamaiedh, H., 2010. Reuse of marble sludge slime in ceramic industry. Jordan Journal of Civil Engineering, 4(3), Pp. 264-271.
Aljbour, S. H., Al-Hamaiedeh, H., El-Hasan, T., Hayek, B. O., Abu-Samhadaneh, K., Al-Momany, S., and Aburawaa, A., 2021. Anaerobic co-digestion of domestic sewage sludge with food waste: Incorporating food waste as a co-substrate under semi-continuous operation. Journal of Ecological Engineering, 22(7), Pp. 1-10.
Aljbour, S. H., El-Hasan, T., Al-Hamiedeh, H., Hayek, B., and Abu-Samhadaneh, K., 2021. Anaerobic co-digestion of domestic sewage sludge and food waste for biogas production: A decentralized integrated management of sludge in Jordan. Journal of Chemical Technology and Metallurgy, 56(5), Pp. 1030-1038.
Al-Jrajreh, S. S., Al-Hamaiedeh, H., Al-Kheetan, M. J., Jweihan, Y. S., and Aljaafreh, T., 2023. Improvement of ornamental stone wastes as sand replacement in concrete using silane coupling agent. Results in Engineering, 20, 101580.
Al-Nawaiseh, A. R., Aljbour, S. H., Al-Hamaiedeh, H., El-Hasan, T., Hemidat, S., and Nassour, A., 2021. Composting of Organic Waste: A Sustainable Alternative Solution for Solid Waste Management in Jordan. Jordan Journal of Civil Engineering, 15(3).
Al-Qodah, Z., Al-Zghoul, T. M., and Jamrah, A., 2024. The performance of pharmaceutical wastewater treatment system of electrocoagulation assisted adsorption using perforated electrodes to reduce passivation. Environmental Science and Pollution Research, Pp. 1-15.
Al-Zghoul, T. M., Al-Qodah, Z., and Al-Jamrah, A., 2023. Performance, Modeling, and Cost Analysis of Chemical Coagulation-Assisted Solar Powered Electrocoagulation Treatment System for Pharmaceutical Wastewater. Water, 15(5), Pp. 980.
APHA Awwa, W.P.C.F., 2005. Standard methods for the examination of water and wastewater. APHA WEF AWWA.
Arabeyyat, O. S., Shatnawi, N., Shbool, M. A., and Al Shraah, A., 2024. Landfill site selection for sustainable solid waste management using multiple-criteria decision-making. Case study: Al-Balqa governorate in Jordan. MethodsX, Pp. 102591.
Asopa, K. R., and Korake, S. R., 2019. Use of sapodilla seed and dolichos lablab in treatment of grey water. IJRESM, 2, Pp. 755-759.
Assou, M., El Fels, L., El Asli, A., Fakidi, H., Souabi, S., Hafidi, M., 2016. Landfill leachate treatment by a coagulation–flocculation process: Effect of the introduction order of the reagents. Desalin. Water Treat, 57, Pp. 21817–21826.
Azis, A., Yusuf, H., Faisal, Z. and Suradi, M., 2015. Water turbidity impact on discharge decrease of groundwater recharge in recharge reservoir. Procedia Engineering. 125: Pp. 199-206.
Aziz, H. A., Yii, Y. C., Syed Zainal, S. F. F., Ramli, S. F., and Akinbile, C. O., 2018. Effects of using Tamarindus indica seeds as a natural coagulant aid in landfill leachate treatment. Global NEST Journal, 20(2), Pp. 373-380.
Babalola, A., and Busu, I., 2011. Selection of landfill sites for solid waste treatment in Damaturu Town-using GIS techniques. Journal of Environmental Protection, 2(01), Pp. 1.
Banch, T. J., Hanafiah, M. M., Alkarkhi, A. F., and Abu Amr, S. S., 2019. Factorial design and optimization of landfill leachate treatment using tannin-based natural coagulant. Polymers, 11(8), Pp. 1349.
Bashir, M.J., Aziz, H.A., Amr, S.S.A., Sethupathi, S.A.P., Ng, C.A., Lim, J.W., 2015. The competency of various applied strategies in treating tropical municipal landfill leachate. Desalin. Water Treat. 54, Pp. 2382–2395.
Bhalla, B., Saini, M.S., and Jha, M.K., 2013. Effect of age and seasonal variations on leachate characteristics of municipal solid waste landfill. International Journal of Research in Engineering and Technology, 2(8), Pp.223-232.
Bilgili, M.; Demir, A.; Özkaya, B. Influence of leachate recirculation on aerobic and anaerobic decomposition of solid wastes. J. Hazard. Mater. 2007, 143, Pp. 177–183.
Bouchareb, R., Isik, Z., Ozay, Y., Karagunduz, A., Keskinler, B., Dizge, N., 2022. A hybrid process for leachate wastewater treatment: Evaporation and reverse osmosis/sequencing batch reactor. Water Environ. Res. 94, e10717.
Cao, H. T., Nguyen, N. T. T., Anh, P. N., Vu, H. H., and Nguyen, H. T., 2021. Insight into removal TOC and NH4+ from mature landfill leachate using coupled bio-coagulation from Moringa oleifera seeds and ZVI/H2O2 process. Journal of Water Process Engineering, 42, 102112.
Castañeda-Díaz, J., Pavón-Silva, T., Gutiérrez-Segura, E., Colín-Cruz, A., 2017 Electrocoagulation-adsorption to remove anionic and cationic dyes from aqueous solution by PV-energy. Journal of Chemistry 2017.
Chaouki, Z., Mrabet, I. el, Khalil, F., Ijjaali, M., Rafqah, S., and Anouar, S., 2017. Journal of Materials and Environmental Sciences. 8 (8) : Pp. 2781–2791.
Cheng, S. Y., Show, P. L., Juan, J. C., Ling, T. C., Lau, B. F., Lai, S. H., and Ng, E. P., 2020. Sustainable landfill leachate treatment: Optimize use of guar gum as natural coagulant and floc characterization. Environmental research, 188, Pp. 109737.
Chua, S. C., Malek, M. A., Chong, F. K., Sujarwo, W., and Ho, Y. C., 2019. Red lentil (Lens culinaris) extract as a novel natural coagulant for turbidity reduction: An evaluation, characterization and performance optimization study. Water, 11(8), Pp. 1686.
Da Silva, A.A., Galvão, R.B., Júnior, V.A., 2014. Post-treatment of landfill leachate from Cianorte-PR by upward filtration in gravel followed by adsorption on granular activated carbon. Environ. Forum High. Paul. 10, Pp. 220–233.
Das, S., Lee, S. H., Kumar, P., Kim, K. H., Lee, S. S., and Bhattacharya, S. S., 2019. Solid waste management: Scope and the challenge of sustainability. Journal of cleaner production, 228, Pp. 658-678.
Deng, Y., Zhu, X., Chen, N., Feng, C., Wang, H., Kuang, P., Hu, W., 2020. Review on electrochemical system for landfill leachate treatment: Performance, mechanism, application, shortcoming, and improvement scheme. Sci. Total Environ. 2020, 745, 140768.
Elleuch, L., Messaoud, M., Djebali, K., Attafi, M., Cherni, Y., Kasmi, M., Elaoud, A., Trabelsi, I., Chatti, A., 2020. A new insight into highly contaminated landfill leachate treatment using Kefir grains pre-treatment combined with Ag-doped TiO2 photocatalytic process. J. Hazard. Mater. 382, Pp. 121119.
Elnaklah, R., Alotaibi, B.S., 2023. Do people believe in, green, environment: Evidence from students in educational buildings-Case of study with use in urban planning. In AIP Conference Proceedings, Vol. 2928, No. 1. AIP Publishing.
Fan, H.J., Shu, H.Y., Yang, H.S., Chen, W.C., 2006. Characteristics of landfill leachates in central Taiwan. Sci. Total Environ. 2006, 361, Pp. 25–37.
Fernandes, A., Pacheco, M.J., Ciríaco, L., Lopes, A.J.A.C.B.E., 2015. Review on the electrochemical processes for the treatment of sanitary landfill leachates: Present and future. Appl. Catal. B Environ. 176, Pp. 183–200.
García-Guaita, F., González-García, S., Villanueva-Rey, P., Moreira, M. T., and Feijoo, G., 2018. Integrating urban metabolism, material flow analysis and life cycle assessment in the environmental evaluation of Santiago de Compostela. Sustainable cities and society, 40, Pp. 569-580.
Gautam, S., and Saini, G., 2020. Use of natural coagulants for industrial wastewater treatment. Global Journal of Environmental Science and Management. 6(4) : Pp. 553–578.
Genethliou, C., Tatoulis, T., Charalampous, N., Dailianis, S., Tekerlekopoulou, A.G., Vayenas, D.V., 2023. Treatment of raw sanitary landfill leachate using a hybrid pilot-scale system comprising adsorption, electrocoagulation and biological process. J. Environ. Manag. 330, 117129.
Hamaideh, A., Al-Zghoul, T., Dababseh, N., and Jamrah, A., 2024. Enhancing Water Management in Jordan: A Fresh Tomato Water Footprint Analysis. Jordan Journal of Agricultural Sciences.
Jamrah, A., Al-Zghoul, T.M., and Al-Qodah, Z., 2024. An Extensive Analysis of Combined Processes for Landfill Leachate Treatment. Water, 16(12), Pp. 1640.
Jamrah, A., Al-Zghoul, T.M., and Darwish, M.M., 2023. A comprehensive review of combined processes for olive mill wastewater treatments. Case Studies in Chemical and Environmental Engineering, Pp. 100493.
Jotin, R., Ibrahim, S., Halimoon, N., 2012. Electro coagulation for removal of chemical oxygen demand in sanitary landfill leachate. Int. J. Environ. Sci. 3, Pp. 921.
Katayon, S., Noor, M. M. M., Asma, M., Ghani, L. A., Thamer, A. M., Azni, I., … and Suleyman, A. M., 2006. Effects of storage conditions of Moringa oleifera seeds on its performance in coagulation. Bioresource technology, 97(13), Pp. 1455-1460.
Keyikoglu, R., Karatas, O., Rezania, H., Kobya, M., Vatanpour, V., Khataee, A., 2021. A review on treatment of membrane concentrates generated from landfill leachate treatment processes. Sep. Purif. Technol, 259, Pp. 118182.
Khan, M.A., Khan, R., Al-Zghoul, T.M., Khan, A., Hussain, A., Baarimah, A.O., Arshad, M.A., 2024. Optimizing municipal solid waste management in urban Peshawar: A linear mathematical modeling and GIS approach for efficiency and sustainability. Case Studies in Chemical and Environmental Engineering, 100704.
Kulikowska, D., and Klimiuk, E., 2008. The effect of landfill age on municipal leachate composition. Bioresource technology, 99(13), Pp.5981-5985.
Lester-Card, E., Smith, G., Lloyd, G., and Tizaoui, C., 2023. A green approach for the treatment of oily steelworks wastewater using natural coagulant of Moringa oleifera seed. Bioresource Technology Reports, 22, 101393.
Li, W., Zhou, Q., Hua, T.,2010. Removal of organic matter from landfill leachate by advanced oxidation processes: A review. Int. J. Chem. Eng. 2010, 270532.
Lim, H. K., Ismail, N., Abustan, I., Murshed, M. F., and Ahmad, A., 2012. Treatment of landfill leachate by using lateritic soil as a natural coagulant. Journal of environmental management, 112, Pp. 353-359.
Maiti, S.K., De, S., Hazra, T., Debsarkar, A., Dutta, A., 2016. Characterization of leachate and its impact on surface and groundwater quality of a closed dumpsite–a case study at Dhapa, Kolkata, India. Procedia Environ. Sci. 35, Pp. 391–399.
Matouq, M., Jildeh, N., Qtaishat, M., Hindiyeh, M., and Al Syouf, M. Q., 2015. The adsorption kinetics and modeling for heavy metals removal from wastewater by Moringa pods. Journal of Environmental Chemical Engineering, 3(2), Pp. 775–784.
Mojiri, A., Zhou, J.L., Ratnaweera, H., Ohashi, A., Ozaki, N., Kindaichi, T., Asakura, H., 2021. Treatment of landfill leachate with different techniques: An overview. Water Reuse, 11, Pp. 66–96.
Muhammad, M. M., Hamisu, A. J., and Lawan, M. A., 2020. Comparative study of turbidity removal efficiency of green pea (Cicer arietinum) seed and pod extract for turbid water treatment. Adan Journal Of Agriculture, 1(01), Pp. 104-113.
Nouhi, S., Kwaambwa, H. M., Gutfreund, P., and Rennie, A. R., 2019. Comparative study of flocculation and adsorption behaviour of water treatment proteins from Moringa peregrina and Moringa oleifera seeds. Scientific Reports, 9(1), Pp. 17945.
Oladoja, N. A., 2015. Headway on natural polymeric coagulants in water and wastewater treatment operations. Journal of Water Process Engineering, 6, Pp. 174-192.
Öman, C., Malmberg, M., and Wolf-Watz, C., 2000. Handbok för lakvattenbedömning. RVF Rapport 00, 7.
Pappu, A., Saxena, M., Asolekar, S.R., 2007. Solid wastes generation in India and their recycling potential in building materials. Building and environment. 42(6), Pp. 2311-2320.
Patel, H., and Vashi, R.T., 2013. Comparison of naturally prepared coagulants for removal of COD and colour from textile wastewater global. NEST J., 15(4): Pp. 522-528.
Qureshi, K., Mangi, F., and Bhatti, I., 2016. Development of indigenous Bio-coagulant for Turbidity Removal. Sindh University Research Journal-SURJ. Science Series, 48(3).
Raghu, S. J., and Rodrigues, L. L., 2020. Behavioral aspects of solid waste management: A systematic review. Journal of the Air and Waste Management Association, 70(12), Pp. 1268-1302.
Rahmadyanti, E., Hidajati, N.W., And Saputro, M.S.H., 2021. Effectiveness of Moringa Oleifera as a Natural Coagulant and Adsorbent in Leachate Treatment. EM International, Pp. 747-756.
Ramprasad, C., Sona, K., Afridhi, M., Kumar, R., and Gopalakrishnan, N., 2019. Comparative study on the treatment of landfill leachate by coagulation and electrocoagulation processes. Nature Environment and Pollution Technology. 18(3) : Pp. 845-856.
Rügner, H., Schwientek, M., Beckingham, B., Kuch, B. and Grathwohl, P., 2013. Turbidity as a proxy for total suspended solids (TSS) and particle facilitated pollutant transport in catchments. Environmental earth sciences, 69(2), Pp.373-380.
Shan, T.C., Matar, M., al, Makky, E.A., and Ali, E.N., 2017. The use of Moringa oleifera seed as a natural coagulant for wastewater treatment and heavy metals removal. Applied Water Science. 7 (3) : Pp. 1369–1376.
Sivakumar, D., 2013. Adsorption study on municipal solid waste leachate using Moringa oleifera seed. International Journal of Environmental Science and Technology, 10, Pp. 113-124.
SQ, A., HA, A., MJK, B., 2015. Assessment of various tropical municipal landfill leachate characteristics and treatment opportunities. Glob. Nest J. 17, Pp 1–13.
Suhartini, S., Hidayat, N., and Rosaliana, E., 2013. Influence of powdered Moringa oleifera seeds and natural filter media on the characteristics of tapioca starch wastewater. Int. J. Recycl. Org. Waste Agric., 2: Pp. 1-11.
Sulaiman, M., Umar, D. M., Aliyu, B., and Manan, F. A., 2017. Moringa oleifera seed as alternative natural coagulant for potential application in water treatment : A review Akademia Baru Journal of Advanced Review on Scientific potential application in water treatment : A review. Journal of Advanced Review on Scientific Research, 1(1), Pp. 1–11.
Swar, S.S., Boonnorat, J., Ghimire, A., 2023. Algae-based treatment of a landfill leachate pretreated by coagulation-flocculation. J. Environ. Manag. 342, Pp. 118223.
Swelam, A.A., 2019. Removal comparative study for Cd (II) ions from polluted solutions by adsorption and coagulation techniques using Moringa oleifera seeds. Egyptian journal of chemistry, 62(8), Pp. 1499-1517.
Tatsi, A.A., Zouboulis, A.I., Matis, K.A. and Samaras, P., 2003. Coagulation–flocculation pretreatment of sanitary landfill leachates. Chemosphere, 53(7), Pp.737-744.
Vergara, S. E., and Tchobanoglous, G., 2012. Municipal solid waste and the environment: a global perspective. Annual review of environment and resources, 37, Pp. 277-309.
Vieira, A.M.S., Vieira, M.F., Silva, G.F., Araújo, Á.A., Fagundes-Klen, M.R., Veit, M.T., and Bergamasco, R., 2010. Use of Moringa oleifera seed as a natural adsorbent for wastewater treatment. Water, air, and soil pollution, 206, Pp. 273-281.
Vijayaraghavan, G., Sivakumar, T., and Kumar, A. V., 2011. Application of plant based coagulants for waste water treatment. International Journal of Advanced Engineering Research and Studies, 1(1), Pp. 88-92.
Wijekoon, P., Koliyabandara, P.A., Cooray, A.T., Lam, S.S., Athapattu, B.C., Vithanage, M., 2022. Progress and prospects in mitigation of landfill leachate pollution: Risk, pollution potential, treatment and challenges. J. Hazard. Mater. 421, Pp. 126627.
Yamin, M. Z., 2017. Solid Waste Management in Jordan. Eco MENA, January 3.
Pages | 430-443 |
Year | 2024 |
Issue | 4 |
Volume | 8 |